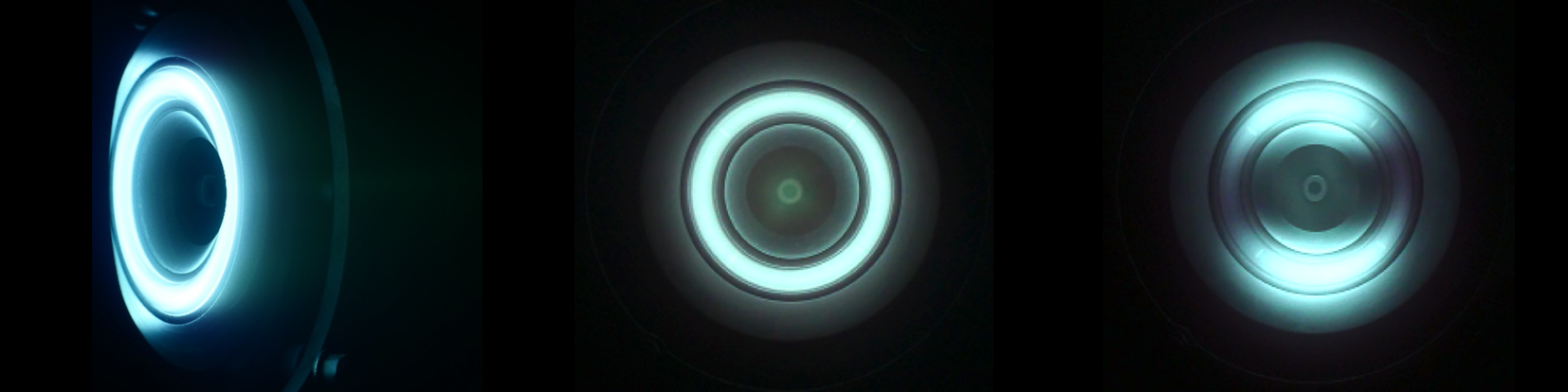
What is a Hall Thruster?
![]() |
Hall Thruster are electric propulsion systems applied on satellites. In Hall thruster, ions are accelerated by the axial electric field applied by the anode, therefore Hall thruster are categorized as electrostatic accelerators, and are hence grouped together with, for example, electrostatic ion thruster. Electrons are trapped by the radial magnetic field and the electric field in the channel of thruster and form circulating Hall current, and that's because this thruster gets its name. Research and development of Hall thruster started already in the 1950s and 60s in the US and the Soviet Union, but US changed the line from Hall thruster to Ion thruster. Soviet union continued the research and development and resulted in the successful application of Hall thruster onboard the Soviet satellite Meteor launched in 1971. Since then research activities were continued especially in the Soviet Union (Russia), where Stationary Plasma Thruster, SPT, were developed and more than 100 SPT have been operated their missions. |
Operation Principle
The Hall thruster has a cylidrical discharge channel, and a magnetic field is applied to the discharge channel in the radial direction by the coils arranged on the central axis and on the outer circumference. Furthermore, an electric field is applied in the axial direction by the anode at the back of the discharge channel and the cathode located downstream of the propulsion unit. The structure of the ExB where the electric field and the magnetic field are orthogonal is the feature of the Hall thrusters. The cathode works as an electron source, so electrons are supplied from the cathode to the discharge channel. Electrons are accelerated toward the anode by the electric field, but are trapped by the magnetic field and move in the circumferential direction by an ExB drift. The movement of electrons in the direction orthogonal to both the electric and magnetic fields is called the Hall effect and this is why the thruster is called a Hall Thruster. The propellant gas is supplied from the anode at the deep inside the discharge channel. Xenon is used for the propellant in many Hall thruster systems. When the molecules of the propellant reach near the exit of the discharge channel where the electrons are particularly strongly trapped, the high energy electrons collide with the propellant molecules and collisional ionization occurs, which knocks out the electrons in the molecules. Molecules from which electrons are taken become positive ions, and are accelerated and exhausted by an electric field applied in the axial direction. The thruster generates thrust by the reaction of force when accelerating these ions. |
![]() |
SPT & TAL
![]() |
There exists two types in Hall thrusters, depending on the configuration. One is the magnetic-layer type (also called stationary plasma thruster, SPT), and this type is the main stream of the Hall thrusters developed in US and Europe. The other one is the anode-layer type (also called thruster with anode layer, TAL), and this type is actively being developed in Japan in the RAIJIN project. SPT and TAL have differences in the thruster configurations as follows: 1) In the discharge channel, SPT has ceramic channel walls, whereas TAL has metallic walls at the cathode potential. 2) SPT generally has the discharge channel length (distance between anode tip and channel exit) of several tens millimeters, while the standard channel length of TAL is smaller than ten millimeter. In Hall thrusters, collisions of the generated ions and electrons with the discharge channel walls mean the energy loss. In TAL, owing to the characteristic 1), the wall electron loss can be minimized (almost zero), and in addition owing to 2), the wall ion loss can also be reduced by accelerating ions within a very short channel. The energy losses in TAL is relatively small, and it is considered that the TAL can achieve a high energy conversion efficiency compared with SPT. |
RAIJIN Project
In recent years, development of high power electric propulsion system has been activated around the world. In addition to the 5 kW class system used as a bus system for the full-electric satellites, 12.5 kW, 20 kW and 50 kW class systems have already been developed in the United States and Europe. The knowledge of the high power electric propulsion system is actively being obtained. In order to develop a unique high power electric propulsion system, Hall thruster researchers at universities in Japan have launched a project called RAIJIN (Robust Anode-layer Intelligent Thruster for Japan IN-space propulsion system). In this project, with the all-Japan research cooperation, we aim to develop a hall thruster with features such as high power, high efficiency, and long life that can contribute to future full-electric satellites and planetary exploration by international cooperation. In the RAIJIN project, instead of SPT, which has become mainstream in Europe and the United States, we are focusing on development of TAL which has a long history of research in Japan. |
![]() |
High-Performance Anode-Layer Thruster Development
In Japan, the thruster with anode layer (TAL) has been investigated for a long time. RAIJIN94 thruster has been developed based on the acquired knowledge of TALs. This thruster has been designed by Kyushu University and University of Tokyo, using many Japan-developed elemental technologires. RAIJIN94 is operated at 5.0 kW, and achieved the maximum thrust efficiency of 0.64 and maximum specific impulse of 2,200 seconds. This thrust performance is competitive with the stationary plasma thrusters (SPTs) of the same power level developed in foreign companies. Further design optimization is persuit including the remaining issue of thruster lifetime. It is expected that a competitive TAL will be developed as the main propulsion for next-generation space missions and staellites systems. In the University of Tokyo, we designed and fabricated the RAIJIN66, which has a 70% outer diameter of the RAJIN94. RAIJIN66 has the scaled discharge channel and magnetic field geometries similar to those of RAIJIN94. A scaling method to maintain the thruster characteristics of TAL is persuit. The technology called magnetic shielding is being applied to TAL for better thruster lifetime. We also perform internal plasma measurements as well as thruster testing. We are conducting our researches by associating thruster performance and plasma physics. |
![]() ![]() |
Azimuthal Physics and Cross-Field Electron Transport
![]() |
Basically, Hall thrusters have an axisymmetric geometry, but there are plasma oscillation phenomena propagating in the azimuthal direction. These plasma oscillations have been concerned as they would be related to the cross-field axial electron transports. The basic principle of Hall thruster is to induce the azimuthal Hall current by the ExB drift of axial electric field and radial magnetic field. Based on the same physics, if an azimuthal electric field is generated by the azimuthal plasma oscillations, an electron flow is induced in the axial direction (toward the anode). The induced electron current in the axial direction results in the increased power consumption. How do the azimuthal plasna oscillations evolve, and how do the azimuthal electric fields affect axial electron transport? Elucidation of these mechanism is necessary for better understanding and performance improvement of Hall thrusters We investigate azimuthal plasma physics and cross-field electron transport from both the numerical simulation and experiment. Numerical and linear stability analyses are used on how azimuthal plasma oscillations are induced. Regarding the effect of azimuthal plasma distribution on axial electron transport, we are doing a unique experiment. Azimuthal inhomogeneity in plasma property is artificially formed by applying azimuthally nonuniform propellant supply or magnetic flux density. The effects of these artificial nonuniformities on azimuthal plasma property and thruster performance are measured. |
Magnetic Field Optimization for Wall Erosion Reduction
Hall thruster uses a magnetic field for trapping electron motions, and this magnetic field has a big influence on the thruster performance. The trapped electrons cannot travel across magnetic field, whereas they can freely move along magnetic lines. Owing to this characteristic, the plasma potential is formed to make the equipotential lines close to magnetic lines, and the electric field directs in the perpendicular of magnetic lines. To prevent ion losses to the channel walls, a lens-shape magnetic field has been employed for Hall thrusters. A technology called "magnetic shielding" has been proposed to use this characteristic effectively. By aligning the magnetic lines with the discharge channel walls, the number of ions colliding with channels walls are drastically decreased. The channel wall erosion due to ion bombardment is one of the main factors limiting the Hall thruster lifetime. The lifetime of Hall thruster would be significantly improved by using the magnetic shielding We conduct a research to apply the magnetic shielding to TALs. TAL has a characteristic that the ion flux flowing to metallic channel walls is measured as current. We are investigating magnetic field and channel configurations that minimize the wall ion fluxes. |
![]() |
Plasma Flow Simulation
![]() |
Modeling technilogies of Hall thruster is being matured, and the Computer Aided Engineering (CAE) is applied in the thruster design processes. Especially, the numerical modeling of plasma flow involves various plasma physics, and the development of plasma simulation code is competitvely conducted around the world to achieve accurate performance prediction. In addition to a standard axisymmetric two-dimensional model for Hall thruster perfromance prediciton, We develop axial-azimuthal two-dimensional model for analyzing azimuthal plasma oscillations and their effects on cross-field electron transport. The plasma flow is modeled by the hybrid model where the ions and neutral atoms are handled by particle method and the electrons are approximated as fluid. By coupling these two-dimensional models, we are trying to achieve a self-consistent simulation of the Hall thruster discharge. |
Vacuum Facility
A large vaccum chamber of 2 m diameter and 3 m length is available. Rotary pump : ULVAC PKS-070, 7,000 l/min × 2 Mechanial booster pump : ULVAC PMB-060B, 103,300 l/min Oil diffusion pump : ULVAC PFL-36, 37,000 l/s Cryopump : ULVAC CRYO-U20H, 10,000 l/s (Ns) Also, this vacuum facility is equiped with shroud panels cooled by liquid nitrogen for space thermal environment. |
![]() |
Dual-Pendulum Thrust Stand
![]() |
A thrust stand measures the thrust generated by plasma thrusters. We developed a dual-pendulum thrust stand which has merits as follows: • Thermal Drift Free: A normal thrust stand has an issue called the thermal drift. The heat from thruster causes a thermal deformation on the stand, generating an error in the thrust measurement. The dual-pendulum thrust stand cancels the effects of thermal deformation between the double pendulums, and the errors from thermal deformation are minimized. • Real-time monitoring: Zero-displacement control is applied to maintain the relative positions of two pendulums by using a electromagnetic actuator. The response time for the control is within 10 seconds, and the thrust can be measured quickly for various conditions. • Portability: The stand system is independent of the vacuum facility, and it can be transported to other vacuum chambers. In fact, we have used this stand for the Hall thruster permance testing in ISAS/JAXA. |
Probe Measurement System
We conduct plasma diagnostics of the Hall thruster discharge plasma inside the discharge channel and in the plume. The obtained data are utilized for thruster design optimization and validation of plasma models. • Faraday Probe : Faraday probe measures the ion beam exhausted from the thruster. The probe is mounted on a movable stage, and the spatial distribution of the ion beam current is obtained by sweeping the stage. • Single Probe : A single probe measures the plasma properties inside or outside of the thruster. A V-I characteristics is obtained by sweeping the voltage of probe surface, and the electron density and electron temperature can be measured. • Emissive Probe : An emissive probe is used for measuring the plasma potential around the thruster. This probe is heated by the applied current. The emissive probe emits thermal electrons, and the floating potential of the probe reaches the plasma potential. Owing to this characteristics, the plasma potential can be measured instantaneously, and the space potential distribution can be obtained in high space resolution. |
![]() |
Journal Articles
Hall Thruster
![]() |
Investigation of correlation between thrust and anode temperature during transient operation of RAIJIN-66. |
Satpathy, Dibyesh, Shu Kawabata, Hokuto Sekine, Rei Kawashima, Kimiya Komurasaki, and Hiroyuki Koizumi: Journal of Electric Propulsion 2(1):5, 2023. | |
![]() |
Wall Ion Loss Reduction by Acceleration Zone Shifting in Anode-Layer Hall Thruster. |
Kawashima, Rei, Yushi Hamada, Shu Kawabata, Kimiya Komurasaki, and Hiroyuki Koizumi: Journal of Propulsion and Power 38(3):489-493, 2022. | |
![]() |
Method of Suppressing Ingestion Particles Flowing Back to a Hall Thruster using a Beam Target during Ground Testing. |
Ito, Gen, Rei Kawashima, Kimiya Komurasaki, and Hiroyuki Koizumi: Transactions of the Japan Society for Aeronautical and Space Sciences 65(4):160-171, 2022. | |
![]() |
A fast convergence fourth-order Vlasov model for Hall thruster ionization oscillation analyses. |
Zhexu, W. A. N. G., Rei Kawashima, and Kimiya Komurasaki: Plasma Science and Technology 24(2):025502, 2021. | |
![]() |
Characterization of acceleration zone shifting in an anode-layer-type Hall thruster RAIJIN66. |
Hamada, Yushi, Rei Kawashima, Junhwi Bak, Kimiya Komurasaki, and Hiroyuki Koizumi: Vacuum 186:110040, 2021. | |
![]() |
Plasma structure and electron cross-field transport induced by azimuthal manipulation of the radial magnetic field in a Hall thruster E × B discharge |
Junhwi Bak, Rei Kawashima, Giuseppe Romanelli, and Kimiya Komurasaki: Journal of Applied Physics, 131(5):053302, 2022. | |
![]() |
Evolution of electron cross-field transport induced by an equilibrium azimuthal electric field in an E × B Hall thruster discharge under an azimuthally inhomogeneous neutral supply |
Junhwi Bak, Rei Kawashima, Jacob Simmonds, and Kimiya Komurasaki: Physics of Plasmas, 28(10):102510, 2021. | |
![]() |
Two-dimensional hybrid model of gradient drift instability and enhanced electron transport in a Hall thruster |
Rei Kawashima and Kimiya Komurasaki: Physics of Plasmas, 28(6):063502, 2021. | |
![]() |
Discharge characteristics and increased electron current during azimuthally nonuniform propellant supply in an anode layer Hall thruster |
Junhwi Bak, Bastiaan Van Loo, Rei Kawashima, and Kimiya Komurasaki: Journal of Applied Physics, 128:023302, 2020. | |
![]() |
Plasma formation and cross-field electron transport induced by azimuthal neutral inhomogeneity in an anode layer Hall thruster |
Junhwi Bak, Rei Kawashima, Kimiya Komurasaki, and Hiroyuki Koizumi: Physics of Plasmas, 26(7):073505, 2019. | |
![]() |
Inflow angular dependence of the capture coefficient in cryopumps |
Gen Ito, Rei Kawashima, Kimiya Komurasaki, and Hiroyuki Koizumi: Vacuum, 160:102-108, 2019. | |
![]() |
High-order upwind and non-oscillatory approach for steady state diffusion, advection–diffusion and application to magnetized electrons |
Amareshwara Sainadh Chamarthi, Kimiya Komurasaki, and Rei Kawashima: Journal of Computational Physics, 374(1):1120-1151, 2018. | |
![]() |
External Discharge Plasma Thruster |
Burak Karadag, Shinatora Cho, Ikkoh Funaki, Yushi Hamada, and Kimiya Komurasaki: Journal of Propulsion and Power, 34(4):1094-1096, 2018. | |
![]() |
Numerical analysis of azimuthal rotating spokes in a crossed-field discharge plasma |
Rei Kawashima, Kentaro Hara, Kimiya Komurasaki: Plasma Sources Science and Technology, 27:035010, 2018. | |
![]() |
Two-dimensional Modeling of the Hall Thruster Discharge with Nonuniform Propellant Supply in Azimuth |
Rei Kawashima, Junhwi Bak, Kimiya Komurasaki, and Hiroyuki Koizumi: Frontier of Applied Plasma Technology, 11(1):7-12, 2018. | |
![]() |
Hall Thruster Development for Japanese Space Propulsion Programs |
Yushi Hamada, Junhwi Bak, Rei Kawashima, Hiroyuki Koizumi, Kimiya Komurasaki, Naoji Yamamoto, Yusuke Egawa, Ikkoh Funaki, Shigeyasu Iihara, Shinatora Cho, Kenichi Kubota, Hiroki Watanabe, Kenji Fuchigami, Yosuke Tashiro, Yuya Takahata, Tetsuo Kakuma, Yusuke Furukubo, Hirokazu Tahara: Transactions of JSASS, 60(5):320-326, 2017. | |
![]() |
A Flux-Splitting Method for Hyperbolic-Equation System of Magnetized Electron Fluids in Quasi-Neutral Plasmas |
Rei Kawashima, Kimiya Komurasaki, and Tony Schönherr: Journal of Computational Physics, 310:202-212, 2016. | |
![]() |
A Hyperbolic-Equation System Approach for Magnetized Electron Fluids in Quasi-Neutral Plasmas |
Rei Kawashima, Kimiya Komurasaki, and Tony Schönherr: Journal of Computational Physics, 284:59-69, 2015. | |
![]() |
Cost Evaluation of In-Space Transportation of a Solar Power Satellite Using OTVs with Hall Thruster Propulsion Systems |
Yuki Ito, Masakatsu Nakano, Tony Schönherr, Shinatora Cho, Kimiya Komurasaki, and Hiroyuki Koizumi: Transactions of Japan Soc. for Aeronautical and Space Sci., Aerospace Technology Japan, 12(ists29):Po_1_7-Po_1_12, 2014. | |
![]() |
Operating Parameters and Oscillation Characteristics of an Anode-Layer Hall Thruster with Argon Propellant |
Daiki Fujita, Rei Kawashima, Yuki Ito, Shohei Akagi, Jun Suzuki, Tony Schönherr, Hiroyuki Koizumi, and Kimiya Komurasaki: Vacuum, 110:159-164, 2014. | |
![]() |
Kinetic Particle Simulation of Discharge and Wall Erosion of a Hall Thruster |
Shinatora Cho, Kimiya Komurasaki, and Yoshihiro Arakawa: Physics of Plasmas, 20(6):063501, 2013. | |
![]() |
Multilayer Coating Method for Investigating Channel-Wall Erosion in a Hall Thruster |
Shinatora Cho, Shigeru Yokota, Kimiya Komurasaki, and Yoshihiro Arakawa: Journal of Propulsion and Power, 29(1):278-281, 2013. | |
![]() |
Japan's Itinerary Towards High-Power Electric Propulsion |
Tony Schönherr, Shinatora Cho, Hiroyuki Koizumi, Kimiya Komurasaki, Naoji Yamamoto, Takeshi Miyasaka, Hitoshi Kuninaka: Proceedings of the MAI, 60, 2012. | |
![]() |
Channel Wall Erosion Modeling of a SPT-Type Hall Thruster |
Shinatora Cho, Shigeru Yokota, Ryotaro Kaneko, Kimiya Komurasaki, and Yoshihiro Arakawa: Transactions of Japan Soc. for Aeronautical and Space Sci., Aerospace Technology Japan, 10(ists28):Pb_25-Pb_30, 2012. | |
![]() |
Magnetic Topology to Stabilize Ionization Oscillation in Anode-layer-type Hall Thruster |
Shigeru Yokota, Daisuke Takahashi, Shinatora Cho, Ryotaro Kaneko, Masaya Hosoda, Kimiya Komurasaki, and Yoshihiro Arakawa: Transactions of Japan Soc. for Aeronautical and Space Sci., Aerospace Technology Japan, 10(ists28):Pb_31-Pb_35, 2012. | |
![]() |
Thrust and Lifetime Performance Evaluation of a 300 W Class SPT and CHT Type Hall Thruster |
Shinatora Cho, Kimiya Komurasaki, Hiroyuki Koizumi, Akira Kobayashi, and Yoshihiro Arakawa: Frontier of Applied Plasma Technology, 5(2):61-66, 2012. | |
![]() |
Effect of Magnetic Field Configuration on Thrust Performance in Anode Layer Type Hall Thruster |
Daisuke Takahashi, Shigeru Yokota, Shinatora Cho, Ryotaro Kaneko, Masaya Hosoda, Kimiya Komurasaki, and Yoshihiro Arakawa: Frontier of Applied Plasma Technology, 4(1):16-19, 2011. | |
![]() |
Diagnosing on Plasma Plume from Xenon Hall Thruster with CR Model |
Juan Yang, Shigeru Yokota, Ryotaro Kaneko, and Kimiya Komurasaki: Physics of Plasmas, 17(10):103504–1–8, 2010. | |
![]() |
Development of Lifetime Evaluation Method Using Multilayer Coating Chip |
Shinatora Cho, Shigeru Yokota, Kentaro Hara, Daisuke Takahashi, Yoshihiro Arakawa, Kimiya Komurasaki, and Akira Kobayashi: Transactions of Japan Soc. for Aeronautical and Space Sci., Aerospace Technology Japan, 8(ists27):Pb_51-Pb_54, 2010. | |
![]() |
Development of Real-time Boron Nitride Erosion Monitoring System for Hall Thrusters by Cavity Ring-Down Spectroscopy |
Naoji Yamamoto, Azer P. Yalin, Lei Tao, Timothy B. Smith, Alec D. Gallimore, and Yoshihiro Arakawa: Transactions of Japan Soc. for Aeronautical and Space Sci., Space Technology Japan, 7(ists26):Pb_1-Pb_6, 2009. | |
![]() |
Influence of Azimuthally Nonuniform Propellant Flow Rate on Thrust Vector and Discharge Current Oscillation in a Hall Thruster |
Yasuhiro Fukushima, Shigeru Yokota, Kimiya Komurasaki, and Yoshihiro Arakawa: Trans. of Japanese Soc. for Aeronautical and Space Sci., Space Technology Japan, 7(ists26):Pb_41-Pb_45, 2009. | |
![]() |
Diagnostics of Xe Ion in an Anode-layer Type Hall Thruster Using Laser-Induced Fluorescence |
Shigeru Yokota, Markus Lempke, Makoto Matsui, Kentaro Hara, Kimiya Komurasaki, and Yoshihiro Arakawa: Trans. of Japanese Soc. for Aeronautical and Space Sci., Space Technology Japan, 7(ists26):Pb_131-Pb_134, 2009. | |
![]() |
Development of Hall Thruster Life Time Measurement Method Using Multilayer Coating Chip |
Shigeru Yokota, Yasuhiro Fukushima, Ryudo Tsukizaki, Kimiya Komurasaki, Yoshihiro Arakawa, and Akira Kobayashi: Frontier of Applied Plasma Technology, 1:83-84, 2008. | |
![]() |
LIF Spectroscopy of a Hall Thruster Plasma Plume |
Markus Lempke, Shigeru Yokota, Makoto Matsui, Kentaro Hara, Kimiya Komurasaki, and Yoshihiro Arakawa: Frontier of Applied Plasma Technology, 1:37-40, 2008. | |
![]() |
Numerical Prediction of Wall Erosion on a Hall Thruster |
Hiroyuki Koizumi, Kimiya Komurasaki, and Yoshihiro Arakawa: Vacuum, 83(1):67-71, 2008. | |
![]() |
Charge Exchange Ion Number Density Distribution in Hall Thruster Plume |
Shigeru Yokota, Daichi Sakoh, Makoto Matsui, Kimiya Komurasaki, and Yoshihiro Arakawa: Vacuum, 83(1):57–60, 2008. | |
![]() |
Development of a Two-Dimensional Dual Pendulum Thrust Stand for Hall thrusters |
Naoki Nagao, Shigeru Yokota, Kimiya Komurasaki, and Yoshihiro Arakawa: Review of Scientific Instruments, 78(11):115108–1–4, 2007. | |
![]() |
Charge Exchange Ions in a Hall Thruster Plume |
Daichi Sakoh, Shigeru Yokota, Kimiya Komurasaki, and Yoshihiro Arakawa: Advances in Applied Plasma Science, 6:65-68, 2007. | |
![]() |
Evaluation Method of Hall Thruster's Lifetime by Using Multilayer Coating |
Yasuhiro Fukushima, Shigeru Yokota, Kimiya Komurasaki, Yoshihiro Arakawa, and Akira Kobayashi: Transaction of JWRI, 36(1):113-114, 2007. | |
![]() |
Suppression of Discharge Current Oscillations in a Hall Thruster |
Naoji Yamamoto, Shigeru Yokota, Keiko Watanabe, Akihiro Sasoh, Kimiya Komurasaki, and Yoshihiro Arakawa: Trans. of Japanese Soc. for Aeronautical and Space Sci., 48(161):169-174, 2005. | |
![]() |
Measurement of Erosion Rate by Absorption Spectroscopy in a Hall Thruster |
Naoji Yamamoto, Shigeru Yokota, Makoto Matsui, Kimiya Komurasaki, and Yoshihiro Arakawa, Review of Scientific Instruments, 76(8):083111, 2005. | |
![]() |
Discharge Current Oscillation in Hall Thrusters |
Naoji Yamamoto, Kimiya Komurasaki, and Yoshihiro Arakawa, Journal of Propulsion and Power, 21(5):870-876, 2005. | |
![]() |
Discharge Plasma Fluctuations in Hall Thrusters |
Naoji Yamamoto, Takafumi Nakagawa, Kimiya Komurasaki, and Yoshihiro Arakawa: Vacuum, 65(3-4):375-381, 2002. | |
![]() |
Optical Measurement of Plasma Oscillations in a Hall Thruster |
Kimiya Komurasaki, and Daisuke Kusamoto: Trans. of Japan Soc. for Aeronautical and Space Sci., 40(130):203-208, 1999. | |
![]() |
Exhaust Beam Profiles of Hall Thrusters |
Daisuke Kusamoto, Kenji Mikami, Kimiya Komurasaki, and Alec D. Gallimore: Trans. of Japan Soc. for Aeronautical and Space Sci., 40(130):238-247, 1998. | |
![]() |
Performance Calculation of Hall Thruster |
Kimiya Komurasaki, and Yoshihiro Arakawa: Acta Astronautica, 38(3):185-192, 1996. | |
![]() |
Two-Dimensional Numerical Model of Plasma Flow in a Hall Thruster |
Kimiya Komurasaki, and Yoshihiro Arakawa: Journal of Propulsion and Power, 11(6):1317-1323 ,1995. | |
![]() |
Hall-Current Ion Thruster Performance |
Kimiya Komurasaki, and Yoshihiro Arakawa: Journal of Propulsion and Power, 8(6):1212-1216, 1992. |
Journal Articles in Japanese
ホールスラスタ
![]() |
推進剤が不一様に供給されたホールスラスタ内のプラズマ諸量 |
朴俊輝,川嶋嶺,水川将暢,小紫公也,小泉宏之: プラズマ応用科学, 25(1):9-14, 2017. | |
![]() |
5 kW級ホールスラスタRAIJINの共同開発 |
小紫公也, 川嶋嶺: 日本航空宇宙学会誌, 65(11):348-353, 2017. | |
![]() |
5kW級ホールスラスタRAIJINの性能解析 |
濱田悠嗣, 朴俊輝, 小紫公也, 江川雄亮, 山本直嗣, 高畑侑弥, 角間 徹生, 古久保裕介, 田原弘一: 日本航空宇宙学会論文集, 65(2):82-86, 2017. | |
![]() |
マグネティックシールディング搭載アノードレイヤ型ホールスラスタの放電室磁場形状改良によるプルーム発散抑制 |
水川将暢, 朴俊輝, 濱田悠嗣, 小紫公也, 川嶋嶺, 小泉宏之: プラズマ応用科学, 24(1):3-8, 2016. | |
![]() |
多層コーティング法に用いるBNの損耗率測定および較正係数の決定 |
細田誠也, 張科寅, 川嶋嶺, 藤田大樹, 伊藤裕樹, Tony Schönherr, 小泉宏之, 小紫公也, 小林明, 荒川義博: プラズマ応用科学, 20(2):126-129, 2012. | |
![]() |
多層コーティング法におけるBNスパッタリング特性について |
細田誠也, 張科寅, 金子亮太郎, 藤田大樹, 荒川義博, 小紫公也: プラズマ応用科学, 19(2):151-154, 2011. | |
![]() |
発光分光法によるホール型推進機の壁面損耗評価 |
金子亮太郎, 横田茂, 張科寅, 原健太郎, 高橋大祐, 小紫公也, 荒川義博: プラズマ応用科学, 18(1):37-42, 2010. | |
![]() |
周方向に非一様な推進剤供給によるホールスラスタの放電安定化 |
福島靖博,横田茂,小紫公也,荒川義博: 日本航空宇宙学会誌, 58(672):8-14, 2010. | |
![]() |
多層コーティング損耗速度計測法の評価 |
張科寅, 横田茂, 福島靖博, 原健太郎, 荒川義博, 小紫公也, 小林明: プラズマ応用科学, 17(2):154-158, 2009. | |
![]() |
多層コーティングチップによる損耗速度計測 |
張科寅, 横田茂, 福島靖博, 原健太郎, 荒川義博, 小紫公也, 小林明: プラズマ応用科学, 17(1):63-66, 2009. | |
![]() |
多層コーティングによるホール型推進機の寿命評価法 |
横田茂,福島靖博,佐孝大地,小紫公也,荒川義博,小林明: プラズマ応用科学, 15(1):61-64, 2007. | |
![]() |
ホールスラスタにおける放電振動の一次元数値解析 |
上田善太郎, 山本直嗣, 小泉宏之, 小紫公也, 荒川義博: 日本航空宇宙学会誌論文集, 55(638):130-134, 2007. | |
![]() |
ホール型推進機プルーム中のキセノン原子数密度測定 |
横田茂, 松井信, 佐孝大地, 小紫公也, 荒川義博: プラズマ応用科学, 14:35-40, 2006. | |
![]() |
ホールスラスタにおける放電振動の一次元数値解析 |
上田善太郎, 山本直嗣, 荒川義博: プラズマ応用科学, 13:69–74, 2005. | |
![]() |
アノードレイヤ型ホールスラスタ内部のシース構造と放電電流振動の数値解析 |
横田茂, 安井伸輔, 熊倉賢, 小紫公也, 荒川義博: 日本航空宇宙学会論文集, 54(632):413-418, 2006. | |
![]() |
ホール型イオン源プルーム中の酸素原子密度計測 |
山本直嗣, 中川貴史, 荒川義博, 松井信, 小紫公也: プラズマ応用科学, 11:29-34, 2003. | |
![]() |
酸素を推進剤に用いたホールスラスタの研究 |
中川貴史, 山本直嗣, 小紫公也, 荒川義博: 日本航空宇宙学会誌, 51(598):606-612, 2003. | |
![]() |
アノードレイヤ型ホールスラスタの作動特性 |
山本直嗣, 中川貴史, 小紫公也, 荒川義博: 日本航空宇宙学会誌, 51(596):492-497, 2003. | |
![]() |
作動ガスに酸素を用いたホール加速器のイオンビーム特性 |
山本直嗣, 中川貴史, 小紫公也, 荒川義博: プラズマ応用科学, 10:30-35, 2002. | |
![]() |
ホール加速器における放電振動と推進性能の関係 |
山本直嗣, 中川貴史, 小紫公也, 荒川義博: プラズマ応用科学, 9:30-35, 2001. | |
![]() |
電気推進の基礎 |
荒川義博: プラズマ・核融合学会誌, 77(8):765-771, 2001. | |
![]() |
ホール型推進機の放電振動 |
山本直嗣, 小紫公也, 荒川義博: プラズマ応用科学, 8:29-36, 2000. | |
![]() |
ホール推進機 |
荒川義博, 小紫公也, 平川美晴: 日本航空宇宙学会誌, 46(530):146-152, 1998. | |
![]() |
粒子モデルを用いた電気推進機プラズマのシミュレーション |
平川美晴, 荒川義博: 日本航空宇宙学会誌, 45(523):444–452, 1997. | |
![]() |
粒子モデルを用いた電気推進機プラズマのシミュレーション |
平川美晴, 荒川義博: 日本航空宇宙学会誌, 45(523):444–452, 1997. | |
![]() |
スーパーエネルギーテクノロジー亜光速ロケットの可能性 |
荒川義博: エネルギー・資源, 14(3):249-255, 1993. | |
![]() |
ホールスラスタの性能とプラズマ加速解析 |
小紫公也, 荒川義博: 日本航空宇宙学会誌, 40(465):46-153, 1992. | |
![]() |
ホールスラスタの一次元プラズマ加速解析 |
平川美晴, 荒川義博: 東京大学工学部紀要, A-29:28-29, 1991. | |
![]() |
ホール型イオンスラスターにおけるビーム加速解析 |
小紫公也, 荒川義博: 東京大学工学部紀要, A-27:28-29, 1989. |